Table of Contents
- 1 Introduction
- 2 Self and Nonself
- 3 The Anatomy of the Immune System
- 4 The Cells and Secretions of the Immune System
- 5 Lymphocytes
- 6 B Cells and Antibodies
- 7 T Cells and Lymphokines
- 8 Natural Killer Cells
- 9 Phagocytes, Granulocytes, and Their Relatives
- 10 Complement
- 11 Mounting an Immune Response
- 12 A Billion Antibodies
- 13 A Web of Idiotypes
- 14 Receptors for Recognizing Antigen
- 15 Immunity, Natural and Acquired
- 16 Vaccines Through Biotechnology
- 17 Disorders of the Immune System: Allergy
- 18 Autoimmune Diseases
- 19 Immune Complex Diseases
- 20 Immunodeficiency Diseases
- 21 Cancers of the Immune System
- 22 Bone Marrow Transplants
- 23 Immunology and Transplants
- 24 Privileged Immunity
- 25 Immunity and Cancer
- 26 The Immune System and the Nervous System
- 27 Frontiers in Immunology: Hybridoma Technology
- 28 The SCID Mouse
- 29 Genetic Engineering
- 30 The Stem Cell
- 31 Glossary
Introduction
The immune system is a complex network of specialized cells and organs that has evolved to defend the body against attacks by “foreign” invaders. When functioning properly it fights off infections by agents such as bacteria, viruses, fungi, and parasites. When it malfunctions, however, it can unleash a torrent of diseases, from allergy to arthritis to cancer to AIDS.
The immune system evolved because we live in a sea of microbes. Like man, these organisms are programmed to perpetuate themselves. The human body provides an ideal habitat for many of them and they try to break in; because the presence of these organisms is often harmful, the body’s immune system will attempt to bar their entry or, failing that, to seek out and destroy them.
The immune system, which equals in complexity the intricacies of the brain and nervous system, displays several remarkable characteristics. It can distinguish between “self” and “nonself.” It is able to remember previous experiences and react accordingly; thus, once you have had chicken pox, your immune system will prevent you from getting it again. The immune system displays both enormous diversity and extraordinary specificity; not only is it able to recognize many millions of distinctive nonself molecules, it can produce molecules and cells to match up with and counteract each one of them. And it has at its command a sophisticated array of weapons.
The success of this system in defending the body relies on an incredibly elaborate and dynamic regulatory-communications network. Millions and millions of cells, organized into sets and subsets, pass information back and forth like clouds of bees swarming around a hive. The result is a sensitive system of checks and balances that produces an immune response that is prompt, appropriate, effective, and self-limiting.
Self and Nonself
At the heart of the immune system is the ability to distinguish between self and nonself. Virtually every body cell carries distinctive molecules that identify it as self.
The body’s immune defenses do not normally attack tissues that carry a self marker. Rather, immune cells and other body cells coexist peaceably in a state known as self-tolerance. But when immune defenders encounter cells or organisms carrying molecules that say “foreign,” the immune troops move quickly to eliminate the intruders.
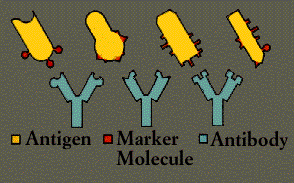
Any substance capable of triggering an immune response is called an antigen. An antigen can be a virus, a bacterium, a fungus, or a parasite, or even a portion or product of one of these organisms. Tissues or cells from another individual, except an identical twin whose cells carry identical self-markers, also act as antigens; because the immune system recognizes transplanted tissues as foreign, it rejects them. The body will even reject nourishing proteins unless they are first broken down by the digestive system into their primary, non-antigenic building blocks.
An antigen announces its foreignness by means of intricate and characteristic shapes called epitopes, which protrude from its surface. Most antigens, even the simplest microbes, carry several different kinds of epitopes on their surface; some may carry several hundred. However, some epitopes will be more effective than others at stimulating an immune response.
In abnormal situations, the immune system can wrongly identify self as nonself and execute a misdirected immune attack. The result can be a so-called autoimmune disease such as rheumatoid arthritis or systemic lupus erythematosus.
In some people, an apparently harmless substance such as ragweed pollen or cat hair can provoke the immune system to set off the inappropriate and harmful response known as allergy; in these cases the antigens are known as allergens.
The Anatomy of the Immune System
The organs of the immune system are stationed throughout the body. They are generally referred to as lymphoid organs because they are concerned with the growth, development, and deployment of lymphocytes, the white cells that are the key operatives of the immune system. Lymphoid organs include the bone marrow and the thymus, as well as lymph nodes, spleen, tonsils and adenoids, the appendix, and clumps of lymphoid tissue in the small intestine known as Peyer’s patches. The blood and lymphatic vessels that carry lymphocytes to and from the other structures can also be considered lymphoid organs.
Cells destined to become immune cells, like all other blood cells, are produced in the bone marrow, the soft tissue in the hollow shafts of long bones. The descendants of some so-called stem cells become lymphocytes, while others develop into a second major group of immune cells typified by the large, cell-and particle-devouring white cells known as phagocytes.
The two major classes of lymphocytes are B cells and T cells. B cells complete their maturation in the bone marrow. T cells, on the other hand, migrate to the thymus, a multilobed organ that lies high behind the breastbone. There they multiply and mature into cells capable of producing immune response-that is, they become immunocompetent. In a process referred to as T cell “education,” T cells in the thymus learn to distinguish self-cells from non-self cells; T cells that would react against self antigens are eliminated.
Upon exiting the bone marrow and thymus, some lymphocytes congregate in immune organs or lymph nodes. Others-both B and T cells-travel widely and continuously throughout the body. They use the blood circulation as well as a bodywide network of lymphatic vessels similar to blood vessels.
Laced along the lymphatic routes-with clusters in the neck, armpits, abdomen, and groin-are small, bean-shaped lymph nodes. Each lymph node contains specialized compartments that house platoons of B lymphocytes, T lymphocytes, and other cells capable of enmeshing antigen and presenting it to T cells. Thus, the lymph node brings together the several components needed to spark an immune response.
The spleen, too, provides a meeting ground for immune defenses. A fist-sized organ at the upper left of the abdomen, the spleen contains two main types of tissue: the red pulp that disposes of worn-out blood cells and the white pulp that contains lymphoid tissue. Like the lymph nodes, the spleen’s lymphoid tissue is subdivided into compartments that specialize in different kinds of immune cells. Microorganisms carried by the blood into the red pulp become trapped by the immune cells known as macrophages. (Although people can live without a spleen, persons whose spleens have been damaged by trauma or by disease such as sickle cell anemia, are highly susceptible to infection; surgical removal of the spleen is especially dangerous for young children and the immunosuppressed.)
Nonencapsulated clusters of lymphoid tissue are found in many parts of the body. They are common around the mucous membranes lining the respiratory and digestive tracts-areas that serve as gateways to the body. They include the tonsils and adenoids, the appendix, and Peyer’s patches.
The lymphatic vessels carry lymph, a clear fluid that bathes the body’s tissues. Lymph, along with the many cells and particles it carries-notably lymphocytes, macrophages, and foreign antigens, drains out of tissues and seeps across the thin walls of tiny lymphatic vessels. The vessels transport the mix to lymph nodes, where antigens can be filtered out and presented to immune cells.
Additional lymphocytes reach the lymph nodes (and other immune tissues) through the bloodstream. Each node is supplied by an artery and a vein; lymphocytes enter the node by traversing the walls of the very small specialized veins.
All lymphocytes exit lymph nodes in lymph via outgoing lymphatic vessels. Much as small creeks and streams empty into larger rivers, the lymphatics feed into larger and larger channels. At the base of the neck, large lymphatic vessels merge into the thoracic duct, which empties its contents into the bloodstream.
Once in the bloodstream, the lymphocytes and other assorted immune cells are transported to tissues throughout the body. They patrol everywhere for foreign antigens, then gradually drift back into the lymphatic vessels, to begin the cycle all over again.
The Cells and Secretions of the Immune System
The immune system stockpiles a tremendous arsenal of cells. Some staff the general defenses, while others are trained on highly specific targets. To work effectively, however, most immune cells require the active cooperation of their fellows. Sometimes they communicate through direct physical contact, sometimes by releasing versatile chemical messengers.
In order to have room for enough cells to match millions of possible foreign invaders, the immune system stores just a few of each specificity. When an antigen appears, those few specifically matched cells are stimulated to multiply into a full-scale army. Later, to prevent this army from overexpanding wildly, like a cancer, powerful suppressor mechanisms come into play.
Lymphocytes
Lymphocytes are small white blood cells that bear the major responsibility for carrying out the activities of the immune system; they number about one trillion. The two major classes of lymphocytes are B cells, which grow to maturity independent of the thymus, and T cells, which are processed in the thymus. Both B cells and T cells recognize specific antigen targets.
B cells work chiefly by secreting soluble substances called antibodies into the body’s fluids, or humors. (This is known as humoral immunity.) Antibodies typically interact with circulating antigens such as bacteria and toxic molecules, but are unable to penetrate living cells. T cells, in contrast, interact directly with their targets, attacking body cells that have been commandeered by viruses or warped malignancy. (This is cellular immunity.)
Although small lymphocytes look identical, even under the microscope, they can be told apart by means of distinctive molecules they carry on their cell surface. Not only do such markers distinguish between B cells and T cells, they distinguish among various subsets of cells that behave differently. Every mature T cell, for instance, carries a marker known as T3 (or CD3); in addition, most helper T cells carry a T4 (CD4) marker, a molecule that recognizes class II MHC antigens. A molecule known as T8 (CD8), which recognizes class I MHC antigens, is found on many suppressor/cytotoxic T cells
In addition, different T cells have different kinds of antigen receptors-either alpha/beta or gamma/delta.
B Cells and Antibodies
Each B cell is programmed to make one specific antibody. For example, one B cell will make an antibody that blocks a virus that causes the common cold, while another produces antibody that zeros in on a bacterium that causes pneumonia.
When a B cell encounters its triggering antigen(along with collaborating T cells and accessory cells), it gives rise to many large plasma cells. Every plasma cell is essentially a factory for producing antibody. Each of the plasma cells descended from a given B cell (which are all members of the same family, or clone) manufactures millions of identical antibody molecules and pours them into the bloodstream.
A given antibody matches an antigen much as a key matches a lock. The fit varies: sometimes it is very precise, while at other times it is little better than that of a skeleton key. To some degree, however, the antibody interlocks with the antigen and thereby marks it for destruction.
Antibodies belong to a family of large molecules known as
immunoglobulins. Immunoglobulins are proteins, made up of chains of polypeptides, strings of the basic units known as amino acids. Each antibody has two identical heavy polypeptide chains and two identical light chains, shaped to form a Y. The sections that make up the tips of the Y’s arms vary greatly from one antibody to another, creating a pocket uniquely shaped to enfold a specific antigen. This is called the variable (V) region. The stem of the Y serves to link the antibody to other participants in the immune defenses. This area is identical in all antibodies of the same class and is called the constant (C) region.
Scientists have identified nine chemically distinct classes of human immunoglobulins (Ig)-four kinds of IgG and two kinds of IgA, plus IgM, IgE, and IgD. Each type plays a different role in the immune defense strategy. IgG, the major immunoglobulin in the blood, is also able to enter tissue spaces; it works efficiently to coat microorganisms, speeding their uptake by other cells in the immune system. IgM, which usually combines in star-shaped clusters, tends to remain in the bloodstream, where it is very effective in killing bacteria. IgA concentrates in body fluids-tears, saliva, the secretions of the respiratory and gastrointestinal tracts-guarding the entrances to the body. IgE, which under normal circumstances occurs only in trace amounts, probably evolved as a defense against parasites, but it is more familiar as the villain in allergic reactions (Allergy). IgD is almost exclusively found inserted into the membranes of B cells, where it somehow regulates the cell’s activation.
Antibodies can work in several ways, depending on the nature of the antigen. Antibodies that interlock with toxins produced by certain bacteria can disable them directly (and are known as antitoxins). Other antibodies, by coating (or opsonizing) bacteria, make the microbes highly palatable to scavenger cells equipped to engulf and destroy them. More often an antigen-antibody combination unleashes a group of lethal serum enzymes known as a complement (Complement). Yet other antibodies block viruses from entering into cells (a quality that is exploited in making vaccines). And, in a phenomenon known as antibody-dependent cell-mediated cytotoxicity (ADCC), cells coated with antibody become vulnerable to attack by several types of white blood cells.
T Cells and Lymphokines
T cells contribute to the immune defenses in two major ways. Regulatory T cells are vital to orchestrating the elaborate system. (B cells, for instance, cannot make antibody against most substances without T cell help). Cytotoxic T cells, on the other hand, directly attack body cells that are infected or malignant.
Chief among the regulatory T cells are “helper/inducer” cells. Typically identifiable by the T4 cell marker, helper T cells are essential for activating B cells and other T cells as well as natural killer cells and macrophages. Another subset of T cells acts to turn off or “suppress” these cells.
B cellCytotoxic T cells, which usually carry the T8 marker, are killer cells. In addition to ridding the body of cells that have been infected by viruses or transformed by cancer, they are responsible for the rejection of tissue and organ grafts. (Although suppressor/ cytotoxic T cells are often called T8 cells, in reality the two are not always synonymous. The T8 molecule, like the T4 molecule, determines which MHC molecule-class I or class II-the T cell will recognize, but not how the T cell will behave.)
T cells work primarily by secreting substances known as
cytokines or, more specifically, lymphokines. Lymphokines (which are also secreted by B cells) and their relatives, the monokines produced by monocytes and macrophages, are diverse and potent chemical messengers. Binding to specific receptors on target cells, lymphokines call into play many other cells and substances, including the elements of the inflammatory response. They encourage cell growth, promote cell activation, direct cellular traffic, destroy target cells, and incite macrophages. A single cytokine may have many functions; conversely, several different cytokines may be able to produce the same effect.
One of the first cytokines to be discovered was interferon. Produced by T cells and macrophages (as well as by cells outside the immune system), interferons are a family of proteins with antiviral properties. Interferon from immune cells, known as immune interferon or gamma interferon, activates macrophages. Two other cytokines, closely related to one another, are lymphotoxin (from lymphocytes) and tumor necrosis factor (from macrophages). Both kill tumor cells; tumor necrosis factor (TNF) also inhibits parasites and viruses.
Many cytokines are initially given descriptive names but, as their basic structure is identified, they are renamed as “interleukins“-messengers between leukocytes, or white cells. Interleukin-1, or IL-1, is a product of macrophages (and many other cells) that helps to activate B cells and T cells. IL-2, originally known as T cell growth factor, or TCGF, is produced by antigen-activated T cells and promotes the rapid growth or differentiation of mature T cells and B cells. IL-3 is a T-cell derived member of the family of protein mediators known as colony-stimulating factors (CSF); one of its many functions is to nurture the development of immature precursor cells into a variety of mature blood cells. IL-4, IL-5, and IL-6 help B cells grow and differentiate; IL-4 also affects T cells, macrophages, mast cells, and granulocytes.
A number of cytokines, obtained in quantity through recombinant DNA technology (Genetic Engineering), are now being used-alone, in combination, linked to toxins-in clinical trials for patients with cancers, blood disorders, and immunodeficiency diseases (including AIDS), as well as people receiving bone marrow transplants. Their versatility, however, makes it difficult to predict the full range of their effects.
Natural Killer Cells
Natural Killer (NK) cells are yet another type of lethal lymphocyte. Like cytotoxic T cells, they contain granules filled with potent chemicals. They are called “natural” killers because they, unlike cytotoxic T cells, do not need to recognize a specific antigen before swinging into action. They target tumor cells and protect against a wide variety of infectious microbes. In several immunodeficiency diseases, including AIDS, natural killer cell function is abnormal. Natural killer cells may also contribute to immunoregulation by secreting high levels of influential lymphokines.
Both cytotoxic T cells and natural killer cells kill on contact. The killer binds to its target, aims its weapons, and then delivers a lethal burst of chemicals that produces holes in the target cell’s membrane. Fluids seep in and leak out, and the cell bursts.
Phagocytes, Granulocytes, and Their Relatives
Phagocytes (literally, “cell eaters”) are large white cells that can engulf and digest marauding microorganisms and other antigenic particles. Some phagocytes also have the ability to present antigen to lymphocytes.
Important phagocytes are monocytes and macrophages. Monocytes circulate in the blood, then migrate into tissues where they develop into macrophages (“big eaters”). Macrophages are seeded throughout body tissues in a variety of guises. Specialized macrophages include alveolar macrophages in the lungs, mesangial phagocytes in the kidneys, microglial cells in the brain, and Kupffer cells in the liver.
Macrophages are versatile cells that play many roles. As scavengers, they rid the body of worn-out cells and other debris. Foremost among the cells that “present” antigen to T cells, having first digested and processed it, macrophages play a crucial role in initiating the immune response. As secretory cells, monocytes and macrophages are vital to the regulation of immune responses and the development of inflammation; they churn out an amazing array of powerful chemical substances (monokines) including enzymes, complement proteins, and regulatory factors such as interleukin-1. At the same time, they carry receptors for lymphokines that allow them to be “activated” into single-minded pursuit of microbes and tumor cells.
Macrophages are not the only cells to present antigen to lymphocytes. Other antigen-presenting cells include B cells, as noted above, and dendritic cells, irregularly shaped white blood cells found in the spleen and other lymphoid organs. Dendritic cells typically have long threadlike tentacles that enmesh lymphocytes and antigens.
Langerhans cells are dendritic cells that travel about in the skin, picking up antigen and transporting it to nearby lymph nodes. Many other types of body cells, properly stimulated, can also be recruited to present antigens to lymphocytes.
Another critical phagocyte is the neutrophil. Neutrophils are not only phagocytes but also granulocytes: they contain granules filled with potent chemicals. These chemicals, in addition to destroying microorganisms, play a key role in acute inflammatory reactions.
Also known as polymorphonuclear leukocytes or polymorphs (because their nuclei come in “many shapes”), granulocytes include eosinophils and basophils as well as neutrophils. (The cells are named for the way they stain in the laboratory: eosinophils, for instance, have an affinity for acidic dyes such as eosin.) The phagocytic neutrophil uses its prepackaged chemicals to degrade the microbes it ingests; eosinophils and basophils typically “degranulate,” releasing their chemicals to work on cells or microbes in their surroundings.
The mast cell is a noncirculating counterpart of the basophil. Located in the lungs, skin, tongue, and linings of the nose and intestinal tract, the mast cell is responsible for the symptoms of allergy (Allergy).
Another related structure is the blood platelet. Platelets, too, contain granules. In addition to promoting blood clotting and wound repair, platelets release substances that activate components of the immune system.
Complement
The complement system is made up of a series of about 25 proteins that work to “complement” the activity of antibodies in destroying bacteria, either by facilitating phagocytosis or by puncturing the bacterial cell membrane. Complement also helps to rid the body of antigen-antibody complexes. In carrying out these tasks, it induces an inflammatory response.
Complement proteins circulate in the blood in an inactive form. When the first of the complement substances is triggered-usually by antibody interlocked with an antigen-it sets in motion ripple effect. As each component is activated in turn, it acts upon the next in a precise sequence of carefully regulated steps known as the “complement cascade.”
In the so-called “classical” pathway of complement activation, a series of proteins gives rise to a complex enzyme capable of cleaving a key protein, C3. In the “alternative” pathway-which can be triggered by suitable targets in the absence of antibody-C3 interacts with a different set of factors and enzymes. But both pathways end in creation of a unit known as the membrane attack complex. Inserted in the wall of the target cell, the membrane attack complex constitutes a channel that allows fluids and molecules to flow in and out. The target cell rapidly swells and bursts.
Meanwhile, various fragments flung off during the course of the cascade can produce other consequences. One byproduct causes mast cells and basophils to release their contents, producing the redness, warmth, and swelling of the inflammatory response. Another stimulates and attract neutrophils. Yet another, C3b, opsonizes or coats target cells so as to make them more palatable to phagocytes, which carry a special receptor for C3b.
The C3b fragment also appears to play a major role in the body’s control of immune complexes. By opsonizing antigen-antibody complexes, C3b helps prevent the formation of large and insoluble (and thus potentially damaging) immune aggregates. Moreover, receptors for C3b are also present on red blood cells, which appear to use the receptors to pick up complement-coated immune complexes and deliver them to the Kupffer cells in the liver.
Mounting an Immune Response
Infections remain the most common cause of human disease. Produced by bacteria, viruses, parasites and fungi, infections may range from relatively mild respiratory illnesses such as the common cold, to debilitating conditions like chronic hepatitis, to life-threatening diseases such as AIDS and meningitis.
To fend off the threatening horde, the body as devised astonishingly intricate defenses. Microbes attempting to enter the body must first find a chink in the body’s external protection. The skin and the mucous membranes that line the body’s portals not only pose a physical barrier, they are also rich in scavenger cells and IgA antibodies.
Next, invaders must elude a series of nonspecific defenses-those cells and substances equipped to tackle infectious agents without regard for their antigenic peculiarities. Many potential infections are cut short when microbes are intercepted by patrolling scavenger cells or disabled by complement or other enzymes or chemicals. Virus-infected cells, for instance, secrete interferon, a chemical that rouses natural killer cells.
A Billion Antibodies
Scientists were long puzzled by the opulence of the immune system’s resources. The body apparently could recognize and mount unique responses to an endless variety of antigens-but how in the world could all that information be crammed into a limited number of genes?
The answer came as a surprise. A typical gene consists of a fixed segment of DNA, which directs the manufacture of a given protein molecule such as insulin. Antibody genes, in contrast, are assembled from bits and pieces of DNA scattered widely throughout the genetic materials. As the B cell matures, it rearranges or shuffles these gene components, picking and choosing among hundreds of DNA segments-some for each of the antibody’s variable (V), diversity (D), joining (J), and constant (C) regions. Intervening segments of DNA are cut out; the selected pieces are spliced together.
The new gene-and the antibody it encodes-are virtually unique. When the B cell containing this uniquely rearranged set of gene segments proliferates, all its descendants will make this unique antibody. Then, as the cells continue to multiply, numerous mutants arise; these allow for the natural selection of antibodies that provide better and better “fits” for the target antigen. The result of this entire process is that a limited number of genetically distinct B cells can respond to a seemingly unlimited range of antigens.
A similar mechanism was found to control a comparable structure of the T cell, the T cell’s antigen receptor. The
variable regions of T cell antigen receptors, like those of antibodies, are encoded by V, D, and J segments originally far apart, but which are brought together and fused into a single gene. With numerous candidates for each segment, the number of possible combinations becomes astronomical. However, in contrast to antibody genes, T cell receptor genes do not mutate as the T cells proliferate. This ensures that the self-tolerance imposed in the thymus will not be overthrown by the inadvertent generation of mutant T cell receptors that are anti-self.
A Web of Idiotypes
The unique and characteristic pocket on an antibody that recognizes a specific antigen-its variable region-can itself act as an antigen. More precisely, the variable region contains a number of antigen-like segments, and these are known collectively as an idiotype. Like any other antigen, an idiotype can trigger complementary antibody. This second-round antibody is known as an antiidiotype. An antiidiotype, in turn, can trigger an antiantiidiotype. Like a series of mirrored reflections, the process can go on and on.
Interactions between idiotypes and antiidiotypes, it has been proposed, constitute a mechanism whereby the immune system regulates itself. According to the “network theory,” not only antibodies but B cells and T cells carry-in their unique antigen-receptors-idiotypes. The B cells and T cells that proliferate in response to a certain antigen carry a complementary idiotype. Antiidiotype B cells secrete antiidiotype antibodies, which may neutralize the original idiotypes (antibodies), or bind to idiotypes on regulatory T cells. Alternatively, antiidiotypes may trigger antiantiidiotypes, creating a spiraling response within the network-turning on, amplifying, and shutting down immune responses.
The concept of the idiotype is being put to practical use today in the development of experimental antigen-free vaccines (Vaccines Through Biotechnology).
Microbes that breach the nonspecific barriers are confronted by specific weapons tailored to fit each one. These may be cellular responses directed both by cells, primarily T lymphocytes and their secretions (lymphokines), and against cells that have been infected. Or they may be humoral responses, the work of antibodies secreted by B lymphocytes into the body’s fluids or humors.
Most antigens are recognized by a limited number of specific immune cells ( and their offspring). A few antigens, however, are capable of rousing large classes of T cells, setting off an immune response so massive that it is harmful. Dubbed ”
superantigens,” these substances include bacterial toxins such as those responsible for the toxic shock syndrome.
Although immunologists traditionally distinguished between cellular and humoral immunity, it has become increasingly clear that the two arms of the immune response are closely intertwined. Almost all antigens evoke both a humoral response and a cellular response-and most B cell responses require T cell help. In practice, however, one arm is usually more effective than the other, and regulatory mechanisms end up skewing the response toward either the cellular or the humoral side.
The cell-mediated response is initiated by a macrophage or other antigen-presenting cell. The antigen-presenting cell takes in the antigen, digests it, and then displays antigen fragments on its own surface. Bound to the antigen fragment is an MHC molecule. It takes both of these structures, together, to capture the T cell’s attention.
A T cell whose receptor fits this antigen-MHC complex binds to it. The binding stimulates the antigen-presenting cell to secrete interleukins required for T cell activation and performance.
Before activated T cells can set to work, however, they need a second go-ahead signal. In a maneuver known as
co-stimulation, the antigen-presenting cell displays a special molecule that engages specific receptor molecules on the T cell, including one known as CD28. Without co-stimulation, activated T cells fall into a state of unresponsiveness known as anergy. Anergy arrests T cell growth by blocking its ability to produce or respond to signals to proliferate.
Once up and going, some subsets of T cells synthesize and secrete lymphokines. Interleukin-2, for instance, spurs the growth of more T cells. Other lymphokines attract other immune cells-fresh macrophages, granulocytes, and other lymphocytes-to the site of the infection. Yet others direct the cells’ activities once they arrive on the scene. Some subsets of T cells become killer (or cytotoxic) cells, and set out to track down body cells infected by viruses. And when the infection has been brought under control, suppressor T cells draw the immune response to a close.
Receptors for Recognizing Antigen
In order to recognize and respond to the antigens that are their specific targets, both B cells and T cells carry special receptor molecules on their surface. For the B cell, this receptor is a prototype of the
antibody
the B cell is prepared to manufacture, anchored in its surface. When a B cell encounters a matching antigen in the blood or other body fluid, this antibody-like receptor allows the B cell to interact with it very efficiently.
The T cell receptor is more complex. Structurally, it is somewhat similar to an antibody, made of a pair of chemically linked chains with variable and
constant regions. (But to work, it needs the help of an associated set of signaling and anchoring cell surface molecules called T3.) Unlike a B cell, however, a T cell cannot recognize antigen in its natural state; the antigen must first be broken down, and the fragments bound to an MHC molecule, by an antigen-presenting cell.
Helper T cells (T4 cells) look for antigen bound to a class II MHC molecule-a combination displayed by macrophages and B cells. Most cytotoxic T cells (T8), on the other hand, respond to antigen bound to MHC class I molecules, which are found on almost all body cells.
The T cell receptor molecule thus forms a three-way complex with its specific foreign antigen and an MHC protein. This complicated arrangement assures that T cells-which affect other cells through either direct contact or bursts of secretions-act only on precise targets and at close range.
The major antigen receptor, name alpha/beta for its two chains, is found on most T4 and T8 cells. A second, more recently discovered antigen receptor also has two chains and is known as gamma/delta; it is found on a distinct subset of mature T cells. Like the alpha/beta receptor, the more primitive gamma/delta receptor works in conjunction with T3. The function of T cells that carry gamma/delta receptors is not known.
Humoral immunity chiefly involves B cells, although the cooperation of helper T cells is almost always necessary. B cells, like macrophages, take in and process circulating antigen. Unlike macrophages, however a B cell can bind only that antigen that specifically fits its antibody-like receptor.
To enlist the help of a T cell, the B cell exhibits antigen fragments bound to its class II MHC molecules. This display attracts mature helper T cells (which may have been already activated by macrophages presenting the same antigen). The B cell and T cell interact, and the helper T cell secretes several lymphokines. These lymphokines set the B cell to multiply, and soon there is a clone of identical B cells. The B cells differentiate into plasma cells and begin producing vast quantities of identical antigen-specific antibodies.
Released into the bloodstream, the antibodies lock onto matching antigens. The antigen-antibody complexes trigger the complement cascade or are removed from the circulation by clearing mechanisms in the liver and the spleen. The infection is overcome and, in response to suppressor influences wielded by yet other subsets of T cells, antibody production wanes.
Clinically, infections manifest themselves through the five classic symptoms of the inflammatory response-redness, warmth, swelling, pain, and loss of function. Redness and warmth develop when, under the influence of lymphokines and complement components, small blood vessels in the vicinity of the infection become dilated and carry more blood. Swelling results when the vessels, made leaky by yet other immune secretions, allow fluid and soluble immune substances to seep into the surrounding tissue, and immune cells to converge on the site.
Immunity, Natural and Acquired
As long ago as the 5th century B.C., Greek physicians noted that people who had recovered from the plague would never get it again-they had acquired immunity. This is because, whenever T cells and B cells are activated, some of the cells become “memory” cells. Then, the next time that an individual encounters that same antigen, the immune system is primed to destroy it quickly.
The degree and duration of immunity depend on the kind of antigen, its amount, and how it enters the body. An immune response is also dictated by heredity; some individuals respond strongly to a given antigen, others weakly, and some not at all.
Infants are born with relatively weak immune responses. They have, however, a natural “passive” immunity; they are protected during the first months of life by means of antibodies they receive from their mothers. The antibody IgG, which travels across the placenta, makes them immune to the same microbes to which their mothers are immune. Children who are nursed also receive IgA from breast milk; it protects the digestive tract.
Types of immunity
Passive immunity
can also be conveyed by antibody-containing serum obtained from individuals who are immune to a specific infectious agent. Immune serum globulin or “gamma globulin” is sometimes given to protect travelers to countries where hepatitis is widespread. Passive immunity typically lasts only a few weeks.
“Active” immunity-mounting an immune response can be triggered by both infection and vaccination. Vaccines contain microorganisms that have been altered so they will produce an immune response but will not be able to induce full-blown disease. Some vaccines are made from microbes that have been killed. Others use microbes that have been changed slightly so they can no longer produce infection. They may, for instance, be unable to multiply. Some vaccines are made from a live virus that has been weakened, or attenuated, by growing it for many cycles in animals or cell cultures.
Recent research, benefiting from the biotechnology revolution, has focused on developing vaccines that use only part of the infectious agent. Such subunit vaccines, which are now available for meningitis, pneumonia, and hepatitis B, produce the desired immunity without stirring up separate and potentially harmful immune reactions to the many antigens carried, for instance, on a single bacterium.
Vaccines Through Biotechnology
Through genetic engineering, scientists can isolate specific genes and insert them into DNA of certain microbes or mammalian cells; the microbes or cells become living factories, mass producing the desired antigen. Then, using another product of biotechnology, a monoclonal antibody that recognizes the antigen, the scientists can separate the antigen from all the other material produced by the microbe or cell. This technique has been used to produce immunogenic but safe segments of the hepatitis B virus and the malaria parasite.
In another approach, scientists have inserted genes for desired antigens into the DNA of the vaccinia virus, the large cowpox virus familiar for its role in smallpox immunization. When the reengineered vaccinia virus is inoculated, it stimulates an immune reaction to both the vaccinia and the products of its passenger genes. These have included, in animal experiments, genes from the viruses that cause hepatitis B, influenza, rabies, and AIDS.
Instead of adding a gene, some scientists have snipped a key gene out of an infectious organism. Thus crippled, the microbe can produce immunity but not disease. This technique has been tried with a bacterium that causes the severe diarrheal disease cholera; such a vaccine is commercially available against a virus disease of pigs.
A totally different approach to vaccine development lies in chemical synthesis. Once scientists have isolated the gene that encodes an antigen, they are able to determine the precise sequence of amino acids that make up the antigen. They then pinpoint small key areas on the large protein molecule, and assemble it chemical by chemical. Wholly synthetic vaccines are being explored for malaria and for the major diarrheal diseases that are so devastating in developing countries.
Another pioneering vaccine strategy exploits antiidiotype antibodies (see A Web of Idiotypes). The original antibody (or idiotype) provokes an antiantibody (or antiidiotype) that resembles the original antigen on the disease-causing organism. The antiidiotype will not itself cause disease, but it can serve as a mock antigen, inducing the formation of antibodies that recognize and block the original antigen. To make such a vaccine, scientists inject animals with a monoclonal antibody (idiotype) against a disease-causing microorganism, then harvest the antiidiotypes produced in response.
Disorders of the Immune System: Allergy
The most common types of allergic reactions-hay fever, some kinds of asthma, and hives-are produced when the immune system response to a false alarm. In a susceptible person, a normally harmless substance-grass pollen or house dust, for example-is perceived as a threat and is attacked.
Such allergic reactions are related to the antibody known as immunoglobulin E. Like other antibodies, each IgE antibody is specific; one reacts against oak pollen, another against ragweed. The role of IgE in the natural order is not known, although some scientists suspect that it developed as a defense against infection by parasitic worms.
The first time an allergy-prone person is exposed to an allergen, he or she makes large amounts of the corresponding IgE antibody. These IgE molecules attach to the surfaces of mast cells (in tissue) or basophils (in the circulation). Mast cells are plentiful in the lungs, skin, tongue, and linings of the nose and intestinal tract.
When an IgE antibody sitting on a mast cell or basophil encounters its specific allergen, the IgE antibody signals the mast cell or basophil to release the powerful chemicals stored within its granules. These chemicals include histamine, heparin, and substances that activate blood platelets and attract secondary cells such as eosinophils and neutrophils. The activated mast cell or basophil also synthesizes new mediators, including prostaglandins and leukotrienes, on the spot.
It is such chemical mediators that cause the symptoms of allergy, including wheezing, sneezing, runny eyes and itching. They can also produce anaphylactic shock, a life-threatening allergic reaction characterized by swelling of body tissues, including the throat, and a sudden fall in blood pressure.
Autoimmune Diseases
Sometimes the immune system’s recognition apparatus breaks down, and the body begins to manufacture antibodies and T cells directed against the body’s own constituents-cells, cell components, or specific organs. Such antibodies are known as autoantibodies, and the diseases they produce are called autoimmune diseases. (Not all autoantibodies are harmful; some types appear to be integral to the immune system’s regulatory scheme.)
Autoimmune reactions contribute to many enigmatic diseases. For instance, autoantibodies to red blood cells can cause anemia, autoantibodies to pancreas cells contribute to juvenile diabetes, and autoantibodies to nerve and muscle cells are found in patients with the chronic muscle weakness known as myasthenia gravis.
Autoantibody known as a rheumatoid factor is common in persons with rheumatoid arthritis.
Persons with systemic lupus erythematosus (SLE), whose symptoms encompass many systems, have antibodies to many types of cells and cellular components. These include antibodies directed against substances found in the cell’s nucleus-DNA, RNA, or proteins-which are known as antinuclear antibodies, or ANAs. These antibodies can cause serious damage when they link up with self antigens to form circulating immune complexes, which become lodged in body tissue and set off inflammatory reactions (Immune Complex Diseases).
Autoimmune diseases affect the immune system at several levels. In patients with SLE, for instance, B cells are hyperactive while suppressor cells are underactive; it is not clear which defect comes first. Moreover, production of IL-2 is low, while levels of gamma interferon are high. Patients with rheumatoid arthritis, who have a defective suppressor T cell system, continue to make antibodies to a common virus, whereas the response normally shuts down after about a dozen days.
No one knows just what causes an autoimmune disease, but several factors are likely to be involved. These may include viruses and environmental factors such as exposure to sunlight, certain chemicals, and some drugs, all of which may damage or alter body cells so that they are no longer recognizable as self. Sex hormones may be important, too, since most autoimmune diseases are far more common in women than in men.
Heredity also appears to play a role. Autoimmune reactions, like many other immune responses, are influenced by the genes of the MHC. A high proportion of human patients with autoimmune disease have particular histocompatibility types. For example, many persons with rheumatoid arthritis display the self-marker known as HLA-DR4.
Many types of therapies are being used to combat autoimmune diseases. These include corticosteroids, immunosuppressive drugs developed as anticancer agents, radiation of the lymph nodes, and plasmapheresis, a sort of “blood washing” that removes diseased cells and harmful molecules from the circulation.
Immune Complex Diseases
Immune complexes are clusters of interlocking antigens and antibodies. Under normal conditions immune complexes are rapidly removed from the bloodstream by macrophages in the spleen and Kupffer cells in the liver. In some circumstances, however, immune complexes continue to circulate. Eventually they become trapped in the tissues of the kidneys, lung, skin, joints, or blood vessels. Just where they end up probably depends on the nature of the antigen, the class of antibody-IgG, for instance, instead of IgM-and the size of the complex. There they set off reactions that lead to inflammation and tissue damage.
Immune complexes work their damage in many diseases. Sometimes, as is the case with malaria and viral hepatitis, they reflect persistent low-grade infections. Sometimes they arise in response to environmental antigens such as the moldy hay that causes the disease known as farmer’s lung. Frequently, immune complexes develop in autoimmune disease, where the continuous production of autoantibodies overloads the immune complex removal system.
Immunodeficiency Diseases
Lack of one or more components of the immune system results in immunodeficiency disorders. These can be inherited, acquired through infection or other illness, or produced as an inadvertent side effect of certain drug treatments.
People with advanced cancer may experience immune deficiencies as a result of the disease process or from extensive anticancer therapy. Transient immune deficiencies can develop in the wake of common viral infections, including influenza, infectious mononucleosis, and measles. Immune responsiveness can also be depressed by blood transfusions, surgery malnutrition, and stress.
Some children are born with defects in their immune systems. Those with flaws in the B cell components are unable to produce antibodies (immunoglobulins). These conditions, known as agammaglobulinemias or hypogammaglobulinemias, leave the children vulnerable to infectious organisms; such disorders can be combated with injections of immunoglobulins.
Other children, whose thymus is either missing or small and abnormal, lack T cells. The resultant disorders have been treated with thymic transplants.
Very rarely, infants are born lacking all the major immune defenses; this is known as severe combined immunodeficiency disease (SCID). Some children with SCID have lived for years in germ-free rooms and “bubbles.” A few SCID patients have been successfully treated with transplants of bone marrow (Bone Marrow Transplants).
The devastating immunodeficiency disorder known as the acquired immunodeficiency syndrome (AIDS)
was first recognized in 1981. Caused by a virus (the human immunodeficiency virus, or
HIV) that destroys T4 cells and that is harbored in macrophages as well as T4 cells, AIDS is characterized by a variety of unusual infections and otherwise rare cancers. The AIDS virus also damages tissue of the brain and spinal cord, producing progressive dementia.
AIDS infections are known as “opportunistic” because they are produced by commonplace organisms that do not trouble people whose immune systems are healthy, but which take advantage of the “opportunity” provided by an immune defense in disarray. The most common infection is an unusual and life-threatening form of pneumonia caused by a one-celled organism (a Protozoa) called Pneumocystis carinii. AIDS patients are also susceptible to unusual lymphomas and Kaposi’s sarcoma, a rare cancer that results from the abnormal proliferation of endothelial cells in the blood vessels.
Some persons infected with the AIDS virus develop a condition known as AIDS-related complex, or ARC, characterized by fatigue, fever, weight loss, diarrhea, and swollen lymph glands. Yet other persons who are infected with the AIDS virus apparently remain well; however, even though they develop no symptoms, they can transmit the virus to others.
AIDS is a contagious disease, spread by intimate sexual contact, by direct inoculation of the virus into the bloodstream, or from mother to child during pregnancy. Most of the AIDS cases in the United States have been found among homosexual and bisexual men with multiple sex partners, and among intravenous drug abusers. Others have involved men who received untreated blood products for hemophilia; persons who received transfusions of inadvertently contaminated blood-primarily before the AIDS virus was discovered and virtually eliminated from the nation’s blood supply with a screening test; the heterosexual partners of persons with AIDS; and children born to infected mothers.
There is presently no cure for AIDS, although the antiviral agent zidovuzine (AZT) appears to hold the virus in check, at least for a time. Many other antiretroviral drugs are being tested, as are agents to bolster the immune system and agents to prevent or treat opportunistic infections. Research on vaccines to prevent the spread of AIDS is also underway.
Cancers of the Immune System
Cells of the immune system, like those of other body systems, can proliferate uncontrollably; the result is cancer. Leukemias are caused by the proliferation of white blood cells, or leukocytes. The uncontrolled growth of antibody-producing (plasma) cells can lead to multiple myeloma. Cancers of the lymphoid organs, known as lymphomas, include Hodgkin’s disease. These disorders can be treated-some of them very successfully-by drugs and/or irradiation.
Bone Marrow Transplants
When the immune response is severely depressed-as the result of inherited defects, cancer therapy, or AIDS-one possible remedy is a transfer of healthy bone marrow. Bone marrow transplants are also used to treat patients with cancers of the blood, the blood-forming organs, and the lymphoid system-the leukemias and lymphomas.
Once in the circulation, transplanted bone marrow cells travel to the bones where the immature cells grow into functioning B and T cells. Like other transplanted tissue, however, bone marrow from a donor must carry self markers that closely match those of the person intended to receive it. This match is essential not only to prevent the transplant from being rejected, but also to fend off a life-threatening situation known as graft-versus-host disease. In graft-versus-host disease, mature T cells from the donor attack and destroy the tissues of the recipient.
To prevent graft-versus-host disease, scientists have developed techniques to “cleanse” the donor marrow of potentially dangerous mature cells. These include chemicals and, more recently, a monoclonal antibody (OKT3) that specifically recognizes and eliminates mature T cells.
For cancer patients who face immunosuppressive therapy but who have no readily matched donor, doctors have used “autologous” transplants: the person’s bone marrow is removed, frozen, and stored until therapy is complete; then the cells are thawed and reinfused.
Immunology and Transplants
Since organ transplantation was introduced over a quarter of a century ago, it has become a widespread remedy for life-threatening disease. Several thousand kidney transplants are performed each year in the United States alone. In addition, physicians have succeeded in transplanting the heart, lungs, liver and pancreas.
The success of a transplant-whether it is accepted or rejected-depends on the stubbornness of the immune system. For a transplant to “take,” the body of the recipient must be made to suppress its natural tendency to get rid of foreign tissue.
Scientists have tackled this problem in two ways. The first is to make sure that the tissue of the donor and the recipient are as similar as possible.
Tissue typing, or histocompatibility testing, involves matching the markers of self on body tissues; because the typing is usually done on white blood cells, or leukocytes, the markers are referred to as
human leukocyte antigens (HLA). Each cell has a double set of six major antigens, designated HLA-A, B, C, and three types of HLA-D-DR, DP, and DQ. (HLA-A, B, and C are the same as the class I antigens encoded by the genes of the major histocompatibility complex; HLA-D region molecules are the class II MHC antigens.)
Each of the HLA antigens exist-in different individuals-in as many as 20 varieties, so that the number of possible HLA types reaches about 10,000. Histocompatibility testing relies on antibodies to determine if a potential organ donor and recipient share two or more HLA antigens, and thus are likely to make a good “match.” The best matches are identical twins; next best are close relatives, especially brothers and sisters.
The second approach to taming rejection is to lull the recipient’s immune system. This can be achieved through a variety of powerful immunosuppressive drugs. Steroids suppress lymphocyte function; the drug cyclosporine holds down the production of the lymphokine interleukin-2, which is necessary for T cell growth. When such measures fail, the graft may yet be saved with a new treatment: OKT3 is a monoclonal antibody that seeks out the T3 marker carried on all mature T cells. By either destroying T cells or incapacitating them, OKT3 can bring an acute rejection crisis to a halt.
Not surprisingly, any such all-out assault on the immune system leaves a transplant recipient susceptible to both opportunistic infections and lymphomas. Although such patients need careful medical followup, many of them are able to lead active and essentially normal lives.
Privileged Immunity
A child developing in the womb carries foreign antigens from its father as well as immunologically compatible self antigens from its mother, and might be expected to trigger a graft rejection. But the uterus is an “immunologically privileged” site where immune responses are subdued. One source of protection appears to be a substance produced by the child, perhaps in response to antibodies from the mother. The substance promotes the development of special white blood cells in the uterus, and these cells release a factor that blocks the actions of IL-2. Another substance, produced by the uterus, helps disguise antigens on the fetal surface of the placenta, shielding them from the mother’s immune defenses.
Immunity and Cancer
The immune system provides one of the body’s main defenses against cancer. When normal cells turn into cancer cells, some of the antigens on their surface change. These new or altered antigens flag immune defenders, including cytotoxic T cells, natural killer cells, and macrophages.
According to one theory, patrolling cells of the immune system provide continuing bodywide surveillance, spying out and eliminating cells that undergo malignant transformation. Tumors develop when the surveillance system breaks down or is overwhelmed. Some tumors may elude the immune defenses by hiding or disguising their tumor antigens. Alternatively, tumors may survive by encouraging the production of suppressor T cells; these T cells act as the tumor’s allies, blocking cytotoxic T cells that would normally attack it.
Blood tests show that people can develop antibodies to many types of tumor antigens (although the antibodies may not actually be effective in fighting the tumor). Skin testing (similar to skin testing for tuberculosis) has demonstrated that tumors provoke cellular immunity as well. Furthermore, studies indicated that cancer patients have a better prognosis when their tumors are infiltrated with many immune cells. Immune responses may underlie the spontaneous disappearance of some cancers.
Tests using antibodies derived from batches of human serum can detect various tumor-associated antigens-including carcinoembryonic antigen (CEA) and alphafetoprotein (AFP)-in blood samples. Because such antigens develop not only in cancer but in other diseases as well, the antibody tests are not useful for cancer screening in the general population. They are however, valuable in monitoring the course of disease and the effectiveness of treatment in patients known to have cancer.
Scientists have developed monoclonal antibodies (Hybridoma Technology) that are targeted specifically at tumor antigens. Linked to radioactive substances, these antibodies can be used to track down and reveal hidden cancer metastases within the body. Monoclonal antitumor antibodies are also being used experimentally to treat cancer-either in their native form or as immunotoxins, linked to natural toxins, anticancer drugs, or radioactive substances.
Other efforts to attack cancer through the immune system center on stimulating or replenishing the patient’s immune responses with substances known as biological response modifiers. Among these are interferons (now obtained through genetic engineering) and interleukins. In some cases biological response modifiers are injected directly into the patient; in other cases they are used in the laboratory to transform some of the patient’s own lymphocytes into tumor-hungry cells known as lymphokine-activated killer (LAK) cells and tumor-infiltrating lymphocytes (TILS), which are then injected back into the patient. Researchers are even using structures from the tumor cells themselves to construct custom-made anticancer “vaccines.”
The Immune System and the Nervous System
A new field of research, known as psychoneuroimmunology, is exploring how the immune system and the brain may interact to influence health. For years stress has been suspected of increasing susceptibility to various infectious diseases or cancer. Now evidence is mounting that the immune system and the nervous system may be inextricably interconnected.
Research has shown that a wide range of stresses, from losing a spouse to facing a tough examination, can deplete immune resources, causing levels of B and T cells to drop, natural killer cells to become less responsive, and fewer IgA antibodies to be secreted in the saliva.
Biological links between the immune system and the central nervous system exist at several levels. One well-known pathway involves the adrenal glands, which, in response to stress messages from the brain, release corticosteroid hormones into the blood. In addition to helping a person respond to emergencies by mobilizing the body’s energy reserves, these “stress hormones” decrease antibodies and reduce lymphocytes in both number and strength.
More recently, it has become apparent that hormones and neuropeptides (hormone-like chemicals released by nerve cells), which convey messages to other cells of the nervous system and organs throughout the body, also “speak” to cells of the immune system. Macrophages and T cells carry receptors for certain neuropeptides; natural killer cells, too, respond to them. Even more surprising, some macrophages and activated lymphocytes actually manufacture typical neuropeptides. At the same time, some lymphokines, secreted by activated lymphocytes such as interferon and the interleukins, can transmit information to the nervous system. Hormones produced by the thymus, too, act on cells in the brain.
In addition, the brain may directly influence the immune system by sending messages down nerve cells. Networks of nerve fibers have been found that connect to the thymus gland, spleen, lymph nodes, and bone marrow. Moreover, experiments show that immune function can be altered by actions that destroy specific brain areas.
The image that is emerging is of closely interlocked systems facilitating a two-way flow of information, primarily through the language of hormones. Immune cells, it has been suggested, may function in a sensory capacity, detecting the arrival of foreign invaders and relaying chemical signals to alert the brain. The brain, for its part, may send signals that guide the traffic of cells through the lymphoid organs.
Frontiers in Immunology: Hybridoma Technology
Through a stratagem known as hybridoma technology, scientists are now able to obtain, in quantity, substances secreted by cells of the immune system-both antibodies and lymphokines. The ready supply of these materials has not only revolutionized immunology but has also created a resounding impact throughout medicine and industry.
A hybridoma is created by fusing two cells, a secreting cell from the immune system and a long-lived cancerous immune cell, within a single membrane. The resulting hybrid cell can be cloned, producing many identical offspring. Each of these daughter clones will secrete, over a long period of time, the immune cell product. A B-cell hybridoma secretes a single specific antibody.
Such monoclonal antibodies, as they are known, have opened remarkable new approaches to preventing, diagnosing, and treating disease. Monoclonal antibodies are used, for instance, to distinguish subsets of B cells and T cells. This knowledge is helpful not only for basic research but also for identifying different types of leukemias and lymphomas and allowing physicians to tailor treatment accordingly. Quantitating the number of B cells and helper T cells is all-important in immune disorders such as AIDS. Monoclonal antibodies are being used to track cancer antigens and, alone or linked to anticancer agents, to attack cancer metastases. The monoclonal antibody known as OKT3 is saving organ transplants threatened with rejection, and preventing bone marrow transplants from setting off graft-versus-host disease.
Monoclonal antibodies are essential to the manufacture of genetically engineered proteins (Genetic Engineering); they single out the desired protein product so it can be separated from the jumble of molecules surrounding it. monoclonal antibodies are also the key to developing new types of vaccines (Vaccines Through Biotechnology).
With growing experience, scientists have devised several sophisticated variants on the monoclonal antibody. For instance, they have created some monoclonal antibodies of human rather than mouse origin; human monoclonal antibodies can be used for therapy without risking an immune reaction to mouse proteins. They have also succeeded in “humanizing” mouse antibodies by splicing the mouse genes for the highly specific antigen-recognizing portion of the antibody into the human genes that encode the rest of the antibody molecule.
Other monoclonal antibodies have been designed to behave like enzymes; these so-called catalytic antibodies or abzymes speed up, or catalyze, selected chemical reactions by binding to a chemical reactant and holding it in a highly unstable “transition state.” By, in fact, cutting the proteins to which they bind, such antibodies may be useful for such things as dissolving blood clots or destroying tumor cells. Yet other researchers, by fusing two hybridoma cells that produce two different antibodies, have created hybrid hybridomas that secrete artificial antibodies made up of two nonidentical halves. While one arm of the bispecific antibody binds to one antigen, the second arm binds to another. One may bind to a marker molecule, for instance, and the second to a target cell, creating an entirely new way to stain cells. Or, one arm of a chimeric antibody may bind to a killer cell while the other locks to a tumor cell, creating a lethal bridge between the two.
The SCID Mouse
Research in immunology took a giant step forward with the development and manipulation of the SCID mouse. Lacing an enzyme necessary to fashion a functional immune system of their own, SCID mice-like their human counterparts with Severe Combined Immunodeficiency Disease (Immunodeficiency Diseases)-are helpless not only to fight infection but also to reject transplanted tissue.
In the late 1980s, scientists transformed the SCID mouse into an in vivo model of the human immune system. One group of researchers painstakingly transplanted a human fetal thymus gland and lymph nodes into the adult SCID mouse, then injected them with embryonic human immune cells. Some of these cells traveled to the human thymus, where they matured into T cells; others developed into working B cells and macrophages, circulating through the lymph nodes. A second group of researchers implanted mature human T cells in the SCID mouse. Such systems amount to a living test tube, making it possible to study the effects of drugs and of viruses, including HIV, in an intact mammalian immune system.
Genetic Engineering
Genetic engineering, more formally known as recombinant DNA technology, allows scientists to pluck genes (segments of DNA) from one type of organism and combine them with genes of a second organism. In this way, relatively simple organisms such as bacteria or yeast, or even mammalian cells in culture and mammals such as goats and sheep, can be induced to make quantities of human proteins, including hormones such as insulin as well as lymphokines and monokines. Microorganisms can also be made to manufacture proteins from infectious agents such as the hepatitis virus or the AIDS virus, for use in vaccines.
Another facet of recombinant DNA technology involves gene therapy: replacing defective or missing genes with normal genes. The first approved gene therapy trials involved children with severe combined immunodeficiency disease, or SCID (Immunodeficiency Diseases), which is caused by lack of an enzyme due to a single abnormal gene. The missing gene is introduced into a harmless virus, then mixed with progenitor cells from the patient’s bone marrow. When the virus splices its genes into those of the bone marrow cells, it simultaneously inserts the gene for the missing enzyme. Injected back into the patient, the treated marrow cells produce the missing enzyme and revitalize the immune defenses. Researchers are also investigating the use of gene therapy for such diverse conditions as hemophilia, Parkinson’s disease, diabetes, a hereditary form of dangerously high cholesterol, and AIDS.
An increasingly important target for gene therapy is cancer. In pioneering experiments, scientists are removing the immune cell known as the tumor-infiltrating lymphocyte or
TIL
(Immunity and Cancer), or tumor cells themselves, inserting a gene that boosts the cells’ ability to make quantities of a natural anticancer product such as tumor necrosis factor (TNF) or interleukin-2, and then growing the restructured cells in quantity in the laboratory. When the altered cells are returned to the patient, they seek out the tumor and deliver large doses of the anti-cancer chemical. They also appear to mobilize, in some unknown way, additional antitumor defenses.
On the horizon are anticancer vaccines made by manipulating genes. Intended to protect cancer patients against a recurrence, these vaccines can incorporate genes for immunogenic tumor antigens or genes for histocompatibility antigens able to galvanize killer T cells, as well as genes for substances such as TNF or interleukin-2. Other anticancer strategies call for introducing genes that can shut down cancer-promoting oncogenes or replace faulty cancer-restraining suppressor genes.
Genes can be packaged, for delivery, in a variety of ways: inserted into the genetic material of such carriers as the familiar vaccinia virus (Vaccines Through Biotechnology) or inactivated retroviruses, grafted onto a protein carrier that magnifies the immune response (an adjuvant), or tucked into fat globules known as liposomes.
The Stem Cell
Scientists have long sought the hematopoietic stem cell, the precursor cell that continuously replenishes the body’s entire panoply of blood cells, both red and white. Stem cells represent a small portion of all bone marrow cells (perhaps one in 2,000), and they are even rarer in the bloodstream. In the mouse, implanting just a few purified stem cells can completely restore an immune system that has been experimentally destroyed.
Although the human stem cell has yet to be isolated and purified, scientists have discovered that progenitor cells capable of giving rise to an array of blood cells (if not of actually reproducing themselves) carry the cell surface marker CD34. These cells can be sorted out from marrow and blood with monoclonal antibodies that recognize CD34. In experimental programs, CD34 cells are being tested as long-lived vehicles for gene therapy and as an alternative to bone marrow transplants.
Glossary
Acquired immunodeficiency syndrome (AIDS): A life-threatening disease caused by a virus and characterized by a breakdown of the body’s immune defenses.
Active immunity: Immunity produced by the body in response to stimulation by a disease-causing organism or a vaccine.
Agammaglobulinemia: An almost total lack of immunoglobulins, or antibodies.
Allergen: Any substance that causes an allergy.
Allergy: An inappropriate and harmful response of the immune system to normally harmless substances.
Anaphylactic shock: A life-threatening allergic reaction characterized by a swelling of body tissues including the throat, difficulty in breathing, and a sudden fall in blood pressure.
Anergy: A state of unresponsiveness, induced when the T cell’s antigen receptor is stimulated, that effectively freezes T cell responses pending a “second signal” from the antigen-presenting cell (co-stimulation).
Antibody: A soluble protein molecule produced and secreted by B cells in response to an antigen, which is capable of binding to that specific antigen.
Antibody-dependent cell-mediated cytotoxicity (ADCC):An immune response in which antibody, by coating target cells, makes them vulnerable to attack by immune cells.
Antigen: Any substance that, when introduced into the body, is recognized by the immune system.
Antigen-presenting cells: B cells, cells of the monocyte lineage (including macrophages as well as dendritic cells), and various other body cells that “present” antigen in a form that T cells can recognize.
Antinuclear antibody (ANA): An autoantibody directed against a substance in the cell’s nucleus.
Antiserum: Serum that contains antibodies.
Antitoxins: Antibodies that interlock with and inactivate toxins produced by certain bacteria.
Appendix: the Lymphoid organ in the intestine.
Attenuated: Weakened; no longer infectious.
Autoantibody: An antibody that reacts against a person’s own tissue.
Autoimmune disease: A disease that results when the immune system mistakenly attacks the body’s own tissues. Rheumatoid arthritis and systemic lupus erythematosus are autoimmune diseases.
Bacterium: A microscopic organism composed of a single cell. Many but no all bacteria cause disease.
Basophil: A white blood cell that contributes to inflammatory reactions. Along with mast cells, basophils are responsible for the symptoms of allergy.
B cells: Small white blood cells crucial to the immune defenses. Also known as B lymphocytes, they are derived from bone marrow and develop into plasma cells that are the source of antibodies.
Biological response modifiers: Substances, either natural or synthesized, that boost, direct, or restore normal immune defenses. BRMs include interferons, interleukins, thymus hormones, and monoclonal antibodies.
Biotechnology: The use of living organisms or their products to make or modify a substance. Biotechnology includes recombinant DNA techniques (genetic engineering) and hybridoma technology.
Bone marrow: Soft tissue located in the cavities of the bones. The bone marrow is the source of all blood cells.
Cellular immunity: Immune protection provided by the direct action of immune cells (as distinct from soluble molecules such as antibodies).
Chromosomes: Physical structures in the cell’s nucleus that house the genes. Each human cell has 23 pairs of chromosomes.
Clone: (n.)A group of genetically identical cells or organisms descended from a single common ancestor; (v.) to reproduce multiple identical copies.
Complement: A complex series of blood proteins whose action “complements” the work of antibodies. Complement destroys bacteria, produces inflammation, and regulates immune reactions.
Complement cascade: A precise sequence of events usually triggered by an antigen-antibody complex, in which each component of the complement system is activated in turn.
Constant region: That part of an antibody’s structure that is characteristic for each antibody class.
Co-Stimulation: The delivery of a second signal from an antigen-presenting cell to a T cell. The second signal rescues the activated T cell from anergy, allowing it to produce the lymphokines necessary for the growth of additional T cells.
Cytokines: Powerful chemical substances secreted by cells. Cytokines include lymphokines produced by lymphocytes and monokines produced by monocytes and macrophages.
Cytotoxic T cells: A subset of T lymphocytes that can kill body cells infected by viruses or transformed by cancer.
Dendritic cells: White blood cells found in the spleen and other lymphoid organs. Dendritic cells typically use threadlike tentacles to enmesh antigen, which they present to T cells.
DNA (deoxyribonucleic acid): Nucleic acid that is found in the cell nucleus and that is the carrier of genetic information.
Enzyme: A protein, produced by living cells, that promotes the chemical processes of life without itself being altered.
Eosinophil: A white blood cell that contains granules filled with chemicals damaging to parasites, and enzymes that damp down inflammatory reactions.
Epitope: A unique shape or marker carried on an antigen’s surface, which triggers a corresponding antibody response.
Fungus: Member of a class of relatively primitive vegetable organism. Fungi include mushrooms, yeasts, rusts, molds, and smuts.
Gene: A unit of genetic material (DNA) that carries the directions a cell uses to perform a specific function, such as making a given protein.
Graft-versus-host disease (GVHD): A life-threatening reaction in which transplanted immunocompetent cells attack the tissues of the recipient.
Granulocytes: White blood cells filled with granules containing potent chemicals that allow the cells to digest microorganisms, or to produce inflammatory reactions. Neutrophils, eosinophils, and basophils are examples of granulocytes.
Helper T cells: A subset of T cells that typically carry the T4 marker and are essential for turning on antibody production, activating cytotoxic T cells, and initiating many other immune responses.
Hematopoiesis: The formation and development of blood cells, usually takes place in the bone marrow.
Histocompatibility testing: A method of matching the self antigens (HLA) on the tissues of a transplant donor with those of the recipient. The closer the match, the better the chance that the transplant will take.
HIV (human immunodeficiency virus): The virus that causes AIDS.
Human leukocyte antigens (HLA): Protein in markers of self used in histocompatibility testing. Some HLA types also correlate with certain autoimmune diseases.
Humoral immunity: Immune protection provided by soluble factors such as antibodies, which circulate in the body’s fluids or “humors,” primarily serum and lymph.
Hybridoma: A hybrid cell created by fusing a B lymphocyte with a long-lived neoplastic plasma cell, or a T lymphocyte with a lymphoma cell. A B-cell hybridoma secretes a single specific antibody.
Hypogammaglobulinemia: Abnormally low levels of immunoglobulins.
Idiotypes: The unique and characteristic parts of an antibody’s variable region, which can themselves serve as antigens.
Immune complex: A cluster of interlocking antigens and antibodies.
Immune response: The reactions of the immune system to foreign substances.
Immunoassay: A test using antibodies to identify and quantify substances. Often the antibody is linked to a marker such as a fluorescent molecule, a radioactive molecule, or an enzyme.
Immunocompetent: Capable of developing an immune response.
Immunoglobulins: A family of large protein molecules, also known as antibodies.
Immunosuppression: Reduction of the immune responses, for instance by giving drugs to prevent transplant rejection.
Immunotoxin: A monoclonal antibody linked to a natural toxin, a toxic drug, or a radioactive substance.
Inflammatory response: Redness, warmth, swelling, pain, and loss of function produced in response to infection, as the result of increased flood flow and an influx of immune cells and secretions.
Interleukins: A major group of lymphokines and monokines.
Kupffer cells: Specialized macrophages in the liver.
LAK cells: Lymphocytes transformed in the laboratory into lymphokine-activated killer cells, which attack tumor cells.
Langerhans cells: Dendritic cells in the skin that pick up antigen and transport it to lymph nodes.
Leukocytes: All white blood cells.
Lymph: A transparent, slightly yellow fluid that carries lymphocytes, bathes the body tissues, and drains into the lymphatic vessels.
Lymphatic vessels: A bodywide network of channels, similar to the blood vessels, which transport lymph to the immune organs and into the bloodstream.
Lymph nodes: Small bean-shaped organs of the immune system, distributed widely throughout the body and linked by lymphatic vessels. Lymph nodes are garrisons of B, T, and other immune cells.
Lymphocytes: Small white blood cells produced in the lymphoid organs and paramount in the immune defenses.
Lymphoid organs: The organs of the immune system, where lymphocytes develop and congregate. They include the bone marrow, thymus, lymph nodes, spleen, and various other clusters of lymphoid tissue. The blood vessels and lymphatic vessels can also be considered lymphoid organs.
Lymphokines: Powerful chemical substances secreted by lymphocytes. These soluble molecules help direct and regulate the immune responses.
Macrophage: A large and versatile immune cell that acts as a microbe-devouring phagocyte, an antigen-presenting cell, and an important source of immune secretions.
Major histocompatibility complex (MHC): A group of genes that controls several aspects of the immune response. MHC genes code for self-markers on all body cells.
Mast cell: A granule-containing cell found in tissue. The contents of mast cells, along with those of basophils, are responsible for the symptoms of allergy.
Microbes: Minute living organisms, including bacteria, viruses, fungi and protozoa.
Microorganisms: Microscopic plants or animals.
Molecule: The smallest amount of a specific chemical substance that can exist alone. (The break a molecule down into its constituent atoms is to change its character. A molecule of water, for instance, reverts to oxygen and hydrogen.)
Monoclonal antibodies: Antibodies produced by a single cell or its identical progeny, specific for a given antigen. As a tool for binding to specific protein molecules, monoclonal antibodies are invaluable in research, medicine, and industry.
Monocyte: A large phagocytic white blood cell which, when it enters tissue, develops into a macrophage.
Monokines: Powerful chemical substances secreted by monocytes and macrophages. These soluble molecules help direct and regulate the immune responses.
Natural killer (NK) cells: Large granule-filled lymphocytes that take on tumor cells and infected body cells. They are known as “natural” killers because they attack without first having to recognize specific antigens.
Neutrophil: A white blood cell that is an abundant and important phagocyte.
Nucleic acids: Large, naturally occurring molecules composed of chemical building blocks known as nucleotides. There are two kinds of nucleic acids, DNA and RNA.
OKT3: A monoclonal antibody that targets mature T cells.
Opportunistic infection: An infection in an immunosuppressed person caused by an organism that does not usually trouble people with healthy immune systems.
Opsonize: To coat an organism with antibodies or a complement protein so as to make it palatable to phagocytes.
Organism: An individual living thing.
Parasite: A plant or animal that lives, grows and feeds on or within another living organism.
Passive immunity: Immunity resulting from the transfer of antibodies or antiserum produced by another individual.
Peyer’s patches: A collection of lymphoid tissues in the intestinal tract.
Phagocytes: Large white blood cells that contribute to the immune defenses by ingesting microbes or other cells and foreign particles.
Plasma cells: Large antibody-producing cells that develop from B cells.
Platelets: Granule-containing cellular fragments critical for blood clotting and sealing off wounds. Platelets also contribute to the immune response.
Polymorphs: Short for polymorphonuclear leukocytes or granulocytes.
Proteins: Organic compounds made up of amino acids. Proteins are one of the major constituents of plant and animal cells.
Protozoa: A group of one-celled animals, a few of which cause human disease (including malaria and sleeping sickness).
Rheumatoid factor: An autoantibody found in the serum of most persons with rheumatoid arthritis.
RNA (ribonucleic acid): A nucleic acid that is found in the cytoplasm and also in the nucleus of some cells. One function of RNA is to direct the synthesis of proteins.
Scavenger cells: Any of a diverse group of cells that have the capacity to engulf and destroy foreign material, dead tissues, or other cells.
SCID mouse: A laboratory animal that, lacking an enzyme necessary to fashion an immune system of its own, can be turned into a model of the human immune system when injected with human cells or tissues.
Serum: The clear liquid that separates from the blood when it is allowed to clot. This fluid retains any antibodies that were present in the whole blood.
Severe combined immunodeficiency disease (SCID): A life-threatening condition in which infants are born lacking all major immune defenses.
Spleen: A lymphoid organ in the abdominal cavity that is an important center for immune system activities.
Stem cells: Cells from which all blood cells derive. The bone marrow is rich in stem cells.
Subunit vaccine: A vaccine that uses merely one component of an infectious agent, rather than the whole, to stimulate an immune response.
Superantigens: A class of antigens, including certain bacterial toxins, that unleash a massive and damaging immune response.
Suppressor T cells: A subset of T cells that turn off antibody production and other immune responses.
T cells: Small white blood cells that orchestrate and/or directly participate in the immune defenses. Also known as T lymphocytes, they are processed in the thymus and secrete lymphokines.
Thymus: A primary lymphoid organ, high in the chest, where T lymphocytes proliferate and mature.
TIL: Tumor-infiltrating lymphocytes. These immune cells are extracted from the tumor tissue, treated in laboratory, and reinjected into the cancer patient.
Tissue typing: See histocompatibility testing.
Tolerance: A state of nonresponsiveness to a particular antigen or group of antigens.
Tonsils and adenoids: Prominent oval masses of lymphoid tissues on either side of the throat.
Toxins: Agents produced by plants and bacteria, normally very damaging to mammalian cells, that can be delivered directly to target cells by linking them to monoclonal antibodies or lymphokines.
Vaccine: A substance that contains antigenic components from an infectious organism. By stimulating an immune response (but not disease), it protects against subsequent infection by that organism.
Variable region: That part of an antibody’s structure that differs from one antibody to another.
Virus: A Submicroscopic microbe that causes infectious disease. Viruses can reproduce only in living cells.